Development of Basic Coated Shielded Metal Arc Welding Electrode
Meeting AWS: SFA: E 16/8/2 Class with Corrosion and Toughness
Requirements.
1. Introduction
The nominal composition (wt %) of this weld metal is 15.5 Cr, 8.5 Ni,
1.5 Mo. These electrodes are used for welding stainless steels, such as
Types 16-8-2, 316, and 347, for high-temperature, high –pressure
piping systems and for dissimilar welding. The weld deposit generally
has a Ferrite Number in between 0 to 5 FN. The weld deposit produced
with these electrodes has good hot ductility properties that reduces
crater cracking problem even in high-restraint condition. The weld
metal is usable in as welded condition as per codes but it can also be
used in solution-treated condition. The chemical composition of these
electrodes should be balanced very carefully to develop their fullest
properties. In 16Cr-8Ni-1.5Mo properties include excellent high
temperature microstructural stability, high resistance to hot cracking at
very low ferrite (FN) levels, and good cryogenic toughness. Table 1
and Table 2 gives the AWS specification limits for SMAW (MMA)
weld metal.
Table 1 - Chemical composition of E 16-8-2-XX as per AWS SFA5.4
Table 2 - Mechanical properties of E 16-8-2-XX as per AWS SFA 5.4
During the primary phase of consumable development we faced
problem in percentage of elongation as per AWS standard. This
SMAW covered electrode fulfills the requirement of ASME section II
part C, which can be verified with the weld metal chemical
composition and mechanical property as shown in Table 1 and in Table
2. This advanced SMAW covered are characterized by a sophisticated
chemical composition that provides the weld metal with sufficient
impact toughness, Tensile strength with elongation. The Babcock and
Wilcox was the first company that published with reference to 16.8.2
depositing weld metal with about 0.07C-15.6Cr-8.2Ni-1.5Mo. [2] This
composition of weld metal is in the region of austenite „nose‟ of the
Austenite + Martensite (A+M) and Austenite + Ferrite (A+F)
boundaries in the Schaeffler diagram shown in figure 1, assuming 0.04-
0.1%C and constant values of 0.5%Si-1.5%Mn-0.05%N.
However, As per AWS A5.4 and A5.9 state that 16.8.2 weld metal has
usually below 5FN. The box shows that there is a mild chance of
martensite formation in the as-deposited weld metal yet there is no
confirmation that commercial weld metals contain martensite. In 1992
Kotecki corrects the misleading by proposed a new diagram in which
he examined the formation of martensite in composition. He developed
boundaries for 1wt% Mn, 4wt%Mn, and 10 wt% Mn. Since Mn does
not show up in the nickel equivalent of WRC-1992 diagram, a different
limit is required for every Mn level.
As per this new diagram of kotecki martensite-free microstructure of
16.8.2 is observed shown in Figure 2. The lower boundary of 16.8.2
composition box shows there is a probability of as-deposited
martensite. Diagram also includes coefficient of 0.25 for copper in the
nickel equivalent to observe the effect of copper content on ferrite
number.
2. Experimental Work:
Several trials have been taken and established the weld metal chemistry
to improve the low temperature toughness tensile strength and
percentage elongation. The weld metal meeting the chemical
composition requirement is confirmed by optical emission
spectroscopy. The all weld test coupons were prepared by these
electrodes. These test coupons are taken up for characterization and
mechanical property evaluations.
The weld deposit after welding is evaluated with Dye penetration and
X-ray radiography as per ASME Sec V code. Defect free welded joints
were taken for mechanical testing. Once the mechanical properties
evaluation was completed, the weld cross section was cut in order to
characterize the weldment with macro structural analysis for
metallurgical study. For micro structural analysis the specimen was
milled, ground, polished and then etched using the Aqua regia reagent
(15 ml HCL, 5 ml nitric acid) and examined under the microscope.
2.1. Tensile Test
The tensile property of the weld specimen is analyzed using AMSLER
Universal Tensile Testing Machine with a load capacity of 200 kN. The
tensile measurements have been conducted at room temperature (RT).
The round specimens of diameter 12.45 mm and guage length 50 mm
used for tensile testing prepared as per the ASTM standard E-21. The
tensile data is analyzed to estimate the yield strength (YS), ultimate
tensile strength (UTS), total elongation (TE) and RA (%).
2.2. Metallographic Studies
The optical studies have been carried out using optical microscope.
Metallographic specimens have been prepared and cross section area
which is parallel and perpendicular to welding direction were polished
using various grades of emery papers and cloth with fine alumina
particles. This is followed by cleaning with distilled water and
methanol. The aqua regia etchant is used for observing the
microstructure is 65 % HCL and 35% HNO3 solution.
3. Results and Discussion:
In severe applications such as in Petrochemical, Chemical process
plants, Power generation industries a high performance weld metal is
required. The present study contains mechanical properties evaluation
of the high performance SMAW electrode is discussed and challenges
faced during consumable development. The tensile test is performed on
the pure weld deposit at room temperature, resulting values of tensile
test suggests that the tensile strength possessed by the weld specimen is
satisfactory for Petrochemical, Chemical process plants, Power
generation industries applications. From Table 1 it is clear that weld
metal strength is higher than the requirement.
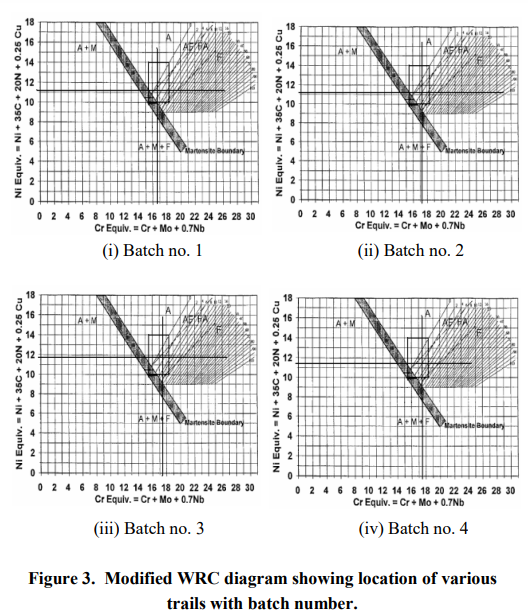
In Figure 3(i) and 3(ii) the composition of weld metal on modified
WRC-1992 diagram is near to martensite boundary zone with around
1%Mn that corresponds closely to as-deposited martensite resulting
lower ductility and brittle fracture even with higher ferrite number
where as if weld metal composition is shifted towards right as shown in
figure 3(iii) and 3(iv) resulting higher ductility and ductile fracture is
observed in tensile test even at very low ferrite number.
Table 4 - Weld deposit composition with various batches
3.1. Tensile Test
The figure shown in 4(a) and 4(b) is representative of brittle fracture
with a very little elongation whereas in figure 4(c) and 4(d) shows
ductile fracture with higher elongation.
The tensile test data as shown in Table 5 are analyzed to evaluate the
ultimate tensile strength (UTS), yield strength (YS), %elongation (EI)
and reduction in area.
It is clear from the tensile test results data that the batches with higher
chromium and nickel content as in batch 3 and batch 4 shows ductile
fracture as compare to batch 1 and batch 2.
3.1. Microstructure
The weld metal microstructure consists with austenite and ferrite in
small proportion and there is no evidence of as-deposited martensite
formation in the weld metal but there should be a possibility of staininduced martensite formation if the composition of weld metal lies
close to the martensite boundary and if carbide precipates during post
weld heat treatment which leads to raise the martensite start
temperature. In figure 5(a) and 5(b) irregularities can be seen, these
irregularities in microstructure resulting brittle fracture or broke off
during tensile testing where as in fig 5(c) and 5(d) uniform
microstructure without irregularities gives ductile fracture in tensile
test. Microstructural stability is obtained by various combination of
composition with low ferrite number as show in figure 5.
3.5 Charpy Impact Testing
For charpy impact testing, the specimens used are cut across the
welded joints having dimensions of 10×10×55 mm and type Vnotched, with 2mm of depth. The charpy impact test is performed as
per ASTM E23 standard to determine the toughness of the material.
Weld metal toughness values at -196°C, -120°C and at -90°C is show
in Table-6.
3.6 Inter-granular corrosion test
Susceptibility of weld metal produced with E-16/8/2 to intergranular
attack is determined by Practice E test as per ASTM-A262 standard.
The test has conducted on as-welded specimen as well as on specimen
after sensitizing heat treatment at 700oC for 30 minutes. The test
specimens are immersed in boiling solution of Cu-Copper Sulfate- 16%
Sulfuric acid for 15 hours. The test procedure is specified in Table 7.
To evaluate micro fissure and cracks 1T bend test has conducted on
specimens after exposed to acidified Cu-CuSO4 H2SO4 test solution.
The bent specimen has examined under 50x magnification is shown in
figure 6.
Metallographic examinations at 50x of the bent specimen showed that
there is no evident of micro fissure and cracks in as-welded specimen
and in specimen after sensitizing heat treatment at 700 deg C.
4. Conclusion:
Basic coated SMAW process consumable was developed in our lab
meeting AWS: SFA: E 16/8/2 -15 class requirements.
Optimum chemistry required for meeting percentage elongation,
corrosion practice E and toughness even at -196°C were formulated.